Contributors
Anthony J. Richardson1,2
Wayne Rochester1
Bronte Tilbrook3,4
1 CSIRO Oceans and Atmosphere, Queensland Biosciences Precinct (QBP), St Lucia, QLD, Australia
2 Centre for Applications in Natural Resource Mathematics (CARM), School of Mathematics and Physics, The University of Queensland, St Lucia, QLD, Australia
3 CSIRO Oceans and Atmosphere, Hobart, TAS, Australia
4 Australian Antarctic Program Partnership, University of Tasmania, Hobart, TAS, Australia Partnership
Key Information
There is no evidence of a decline in calcifying zooplankton at the IMOS National Reference Stations over the past 10 years, suggesting ocean acidification over this time span is unlikely to be having a substantial impact on calcifying zooplankton. However, there is some evidence that calcifying zooplankton might at Maria Island and Yongala be sensitive to the aragonite saturation state at the range of values currently observed.
Keywords
calcareous zooplankton, aragonite saturation state
Ocean acidification and calcifying zooplankton
Download this Time Series Report
Citing this report:
Richardson A.J, Rochester W, Tilbrook B. (2020). Ocean acidification and calcifying zooplankton. The response of the copepod community to long-term warming along the east coast of Australia. In Richardson A.J, Eriksen R, Moltmann T, Hodgson-Johnston I, Wallis J.R. (Eds). State and Trends of Australia’s Ocean Report. doi: 10.26198/5e16b0b249e89
doi: 10.26198/5e16b0b249e89
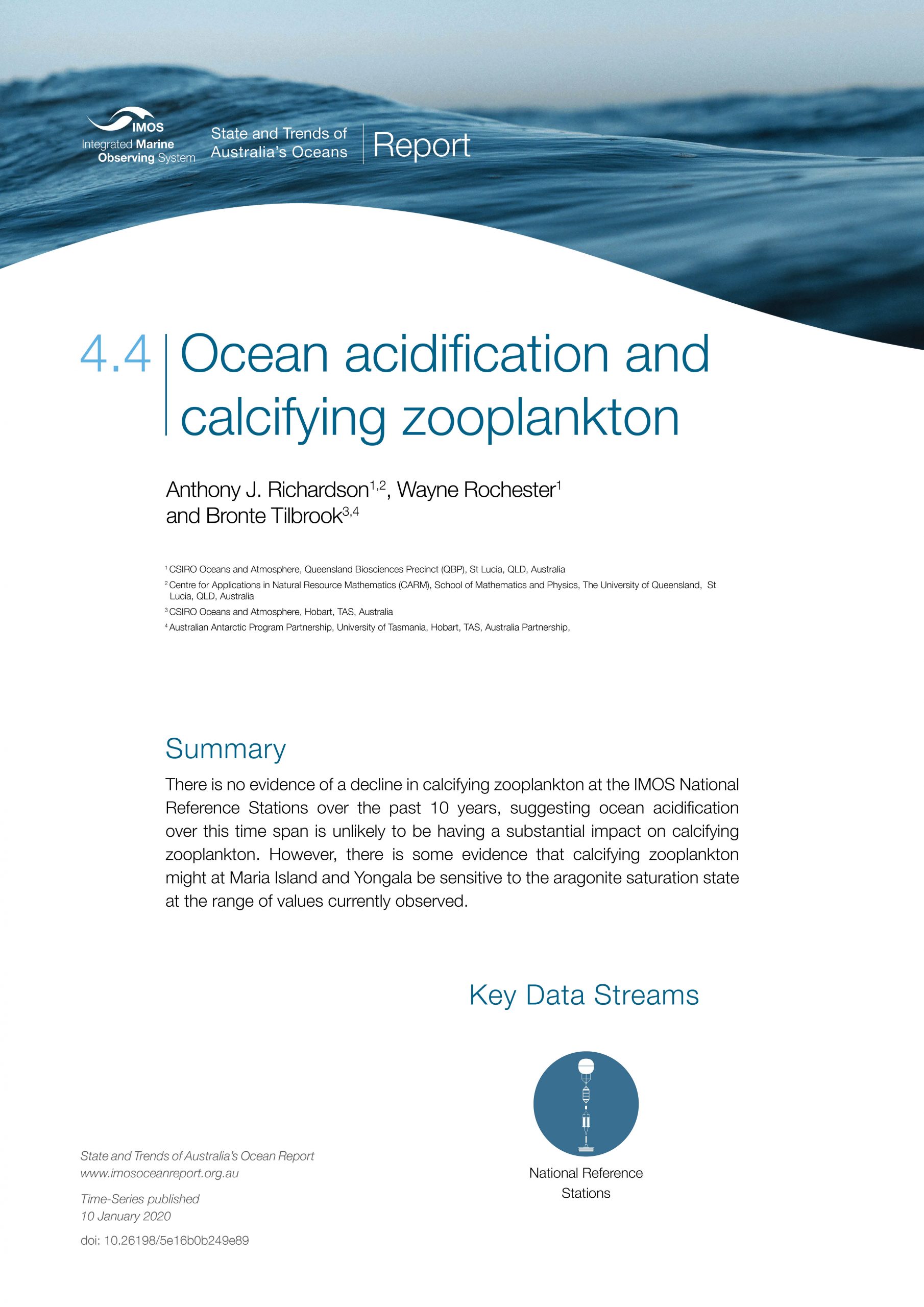