Physical and Chemical
1.3
East Australian Current variability
Contributors
Bernadette M. Sloyan1
Madeleine Cahill1
Moninya Roughan 2
Ken Ridgway1
1 CSIRO Oceans and Atmosphere, Hobart, TAS, Australia
2 School of Mathematics and Statistics, University of New South Wales, Sydney, NSW, Australia
Key Information
The East Australian Current (EAC) is the complex and energetic western boundary current of the South Pacific Ocean, influencing the lives and economies of people on the eastern seaboard. It is the dominant mechanism for the redistribution of heat between the ocean and atmosphere and has a strong influence on the weather and seasonal climate, coastal ocean circulation and marine ecosystem affecting nearly half the Australian population. IMOS’ long-term monitoring of the EAC provides a comprehensive data set that will enable improved understanding and modelling to determine the impact of the EAC on the regional weather and climate, coastal circulation and marine ecosystem.
Keywords
variability frequency, sea surface height anomaly, sea surface temperature anomaly, current velocity
East Australian Current Variability
The East Australian Current (EAC) is the highly energetic western boundary current of the South Pacific Ocean gyre, estimated (27°S) to transport 22.1 +/- 7.5 Sverdrups (Sv; 1 Sv=106m3 s-1) of warm tropical water southward (Sloyan, Ridgway, & Cowley, 2016). Any changes in the EAC in both the short-term or long-term will impact waters along south-eastern Australia – Australia’s most populated coastline – and the Tasman Sea.
Low frequency (>2 year) variability of the EAC reflects changes in the wind and buoyancy forcing over the South Pacific Ocean (Hu et al., 2015). However, local and regional wind and buoyancy forcing drives higher frequency variability (<1-2 years) of the EAC (Bull, Kiss, Jourdain, England, & van Sebille, 2017). Due to the narrow shelf, EAC meandering has an immediate impact on the shelf circulation. Downstream of the EAC separation zone (~30-31.5oS, Cetina-Heredia, Roughan, van Sebille, & Coleman, 2014), mesoscale eddies are shed, which dominate shelf circulation. Exchange of heat, salt and plankton between the shelf and open ocean is achieved via EAC intrusion, submesoscale and mesoscale eddies, and complex boundary layer and frontal dynamics. Therefore, although the EAC transports oligotrophic Coral Sea water, several key processes still stimulate enrichment, including upwelling associated with the dynamics of the EAC (Roughan & Middleton, 2002), mixing associated with frontal and mesoscale eddies (Roughan et al., 2017), and the dynamic exchange of shelf and boundary current water. These enrichment processes influence the entire marine ecosystem, from planktonic production (Armbrecht, Schaeffer, Roughan, & Armand, 2015), and invertebrate and fish larvae to the distribution of large pelagic fish.
To summarise the EAC variability, we combine observations from the Integrated Marine Observing System (IMOS) EAC mooring array and satellite sea surface temperature and velocity at 27°S between May 2015 and April 2018. The IMOS EAC mooring array consists of six moorings in water depths of 500 to 4800 m across 152.94 km, from the continental shelf to the deep abyssal plain. In the upper 1200 m, current profiling instruments provide vertical velocity profiles and below 1200 m, point source velocity instruments provide data at discrete depths. IMOS EAC mooring data were interpolated onto a standard vertical grid and time period and the velocity was rotated to the along-, across-slope coordinate frame. We use the Wijffels et al. (2018) annual mean Sea Surface Temperature (SST) and the night-time satellite sea surface temperature to determine the SST anomaly. Sea surface height (SSH) anomaly was from the IMOS gridded satellite product DM00 GSLA.
A high frequency surface radar system is maintained by IMOS centred at 30°S downstream of the EAC mooring array and upstream of the typical EAC separation region. Surface current data were gridded to 1.5 km resolution over a 100 km square area since 2010. Full details of the radar data and its processing are available from Mantovanelli et al. (2017) . EAC jet core speed and position were calculated from the radar derived current data using the methodology of Archer et al. (2017).
There is considerable variability of SSH and SST between 2015 and 2018 at 27°S (Figure 1). These surface anomalies can extend across the longitudinal extent from the coast to the abyssal basin or partially across the region. There are times when SST and SSH anomalies co-vary, and other times when anomalies are opposed (Figure 1).
To understand the surface anomalies in terms of the EAC, we compare these data with data from the EAC mooring. The mean along-slope velocity vectors show poleward velocity dominates from 60-1500 m. (Figure 2). The strongest southward flow is found over the continental shelf, decreasing in strength in deeper water. The variance ellipses show that the largest variability in EAC transport is in the along-shore direction. This indicates that the EAC variability is dominated by the movement of the EAC on- and off-shore. The EAC thus maintains its jet structure as it meanders onshore and offshore adjacent to the continental slope.
While the mean along-shore velocity vectors provide a picture of the mean 3-year EAC, the time series of velocity show that the EAC has a complex and highly variable structure (Figure 3). The EAC 2000 m mooring is located on the continental slope and measures the EAC core (negative along-slope velocity). The strong southward flow is associated with off-shore flow (positive across-slope velocity). While mostly measuring the EAC core, we see times where the flow is northward (positive along-slope velocity). This northward velocity is due to the shelf flow extending from the coast and is generally associated with on-shore flow (negative across-slope velocity). These changes in the direction and strength of the velocity are driven by cyclonic eddies inshore of the jet, and have significant influence on the exchange between the open ocean and shelf waters.
The Coffs Harbour HF Radar shows that the mean EAC jet lies above the 1500 m isobath, ~50 km offshore (Archer et al., 2017). However, the EAC jet strength is highly variable (Figure 4, top), with maximum surface velocities >2 m/s, and maximum speed in summer and minimum in winter (Archer et al., 2017) . The EAC meanders onshore and offshore adjacent to the continental slope, covering an offshore excursion of >100 km (Figure 4, bottom). The EAC has eastward displacements every 65-100 days, similar to that at 27°S (Sloyan et al., 2016), and is associated with meso-scale eddy shedding at the EAC separation.
The combination of surface and interior observations of the EAC confirm that this current is highly variable and its positions on the shelf influences the exchange between the open and shelf ocean. The interaction between the EAC jet and eddies with prominent topographic features and wind induced upwelling enhances the exchange of nutrient-rich sub-surface water between the open and coastal ocean. These exchanges have a significant influence on the biology of eastern Australian shelf waters and western Tasman Sea.
The long-term EAC mooring arrays at Brisbane (~27°S), Coffs Harbour (~30°S), Sydney (~34°S) and Narooma (~36°S) and the HF radar system at Coffs Harbour provide, unprecedented, comprehensive and valuable information about the EAC and its variability. Although the time series from the EAC mooring array is relatively short currently, over the longer term these observations of temperature and transport will be critical for informing us about how the ocean is responding to climate change, as well as for assessing climate models. The continuation of these observations, coupled with ocean modelling, will improve our understanding of EAC influences on climate, leading to more reliable ocean forecasts for eastern Australia and coastal communities, and help inform management of east coast fisheries.
Archer, M. R., Roughan, M., Keating, S. R., & Schaeffer, A. (2017). On the variability of the East Australian Current: jet structure, meandering, and influence on shelf circulation. Journal of Geophysical Research-Oceans, 122(11), 8464-8481. doi:10.1002/2017jc013097
Armbrecht, L. H., Schaeffer, A., Roughan, M., & Armand, L. K. (2015). Interactions between seasonality and oceanic forcing drive the phytoplankton variability in the tropical-temperate transition zone (similar to 30 degrees S) of Eastern Australia. Journal of Marine Systems, 144, 92-106. doi:10.1016/j.jmarsys.2014.11.008
Bull, C. Y. S., Kiss, A. E., Jourdain, N. C., England, M. H., & van Sebille, E. (2017). Wind forced variability in eddy formation, eddy shedding, and the separation of the East Australian Current. Journal of Geophysical Research-Oceans, 122(12), 9980-9998. doi:10.1002/2017jc013311
Cetina-Heredia, P., Roughan, M., van Sebille, E., & Coleman, M. A. (2014). Long-term trends in the East Australian Current separation latitude and eddy driven transport. Journal of Geophysical Research-Oceans, 119(7), 4351-4366. doi:10.1002/2014jc010071
Hu, D. X., Wu, L. X., Cai, W. J., Sen Gupta, A., Ganachaud, A., Qiu, B., . . . Kessler, W. S. (2015). Pacific western boundary currents and their roles in climate. Nature, 522(7556), 299-308. doi:10.1038/nature14504
Mantovanelli, A., Keating, S., Wyatt, L., Roughan, M., & Schaeffer, A. (2017). Lagrangian and Eulerian characterization of two counterrotating submesoscale eddies in a western boundary current. Geophysical Research Letters, 122(6), 4902-4921.
Roughan, M., Keating, S. R., Schaeffer, A., Heredia, P. C., Rocha, C., Griffin, D., . . . Suthers, I. M. (2017). A tale of two eddies: The biophysical characteristics of two contrasting cyclonic eddies in the East Australian Current System. Journal of Geophysical Research-Oceans, 122(3), 2494-2518. doi:10.1002/2016jc012241
Roughan, M., & Middleton, J. H. (2002). A comparison of observed upwelling mechanisms off the east coast of Australia. Continental Shelf Research, 22(17), 2551-2572. doi:10.1016/s0278-4343(02)00101-2
Sloyan, B. M., Ridgway, K. R., & Cowley, R. (2016). The East Australian Current and property transport at 27 degrees S from 2012 to 2013. Journal of Physical Oceanography, 46(3), 993-1008. doi:10.1175/jpo-d-15-0052.1
Wijffels, S. E., Beggs, H., Griffin, C., Middleton, J. F., Cahill, M., King, E., . . . Sutton, P. (2018). A fine spatial-scale sea surface temperature atlas of the Australian regional seas (SSTAARS): Seasonal variability and trends around Australasia and New Zealand revisited. Journal of Marine Systems, 187, 156-196. doi:10.1016/j.jmarsys.2018.07.005
Figure 1
Hovmöller plots (longitude versus time) of the (left) Sea Surface Height (SSH) anomaly and (right) Sea Surface Temperature (SST) anomaly for a region surrounding the IMOS EAC mooring line (~27°S). Thick black lines identify the location of the IMOS EAC mooring array.
Figure 2
Three-year (May 2015-April 2018) mean along-slope velocity (m/s, green arrow) and velocity variance ellipse (red circle) for (a) 60 m, (b) 120 m, (c) 200 m, and (d) 500 m. The 1000-m, 2000-m, 3000-m, and 4000-m isobaths are contoured and topography shallower than 3000 m is shaded.
Figure 3
Time series (April 2015-May 2018) of the (top) SSH anomaly (m) and SST (°C) anomaly, (middle) Along-slope velocity (m s-1) and (bottom) Across-Slope velocity (m s-1) at ~ 27°S.
Figure 4
(Top) Speed of the core of the EAC jet calculated from HF radar derived surface velocities centered at 30°S, in a flow following framework (Archer et al. 2017) and (bottom) the position of the core of the EAC jet.
Download this Time Series Report
Citing this report:
Sloyan B.M, Cahill M, Roughan M, Ridgway K. (2020). East Australian Current variability. In Richardson A.J, Eriksen R, Moltmann T, Hodgson-Johnston I, Wallis J.R. (Eds). State and Trends of Australia’s Ocean Report. doi: 10.26198/5e16a23f49e75
doi: 10.26198/5e16a23f49e75
Citing the Report
Richardson A.J, Eriksen R, Moltmann T, Hodgson-Johnston I, Wallis J.R. (2020). State and Trends of Australia’s Ocean Report, Integrated Marine Observing System (IMOS).
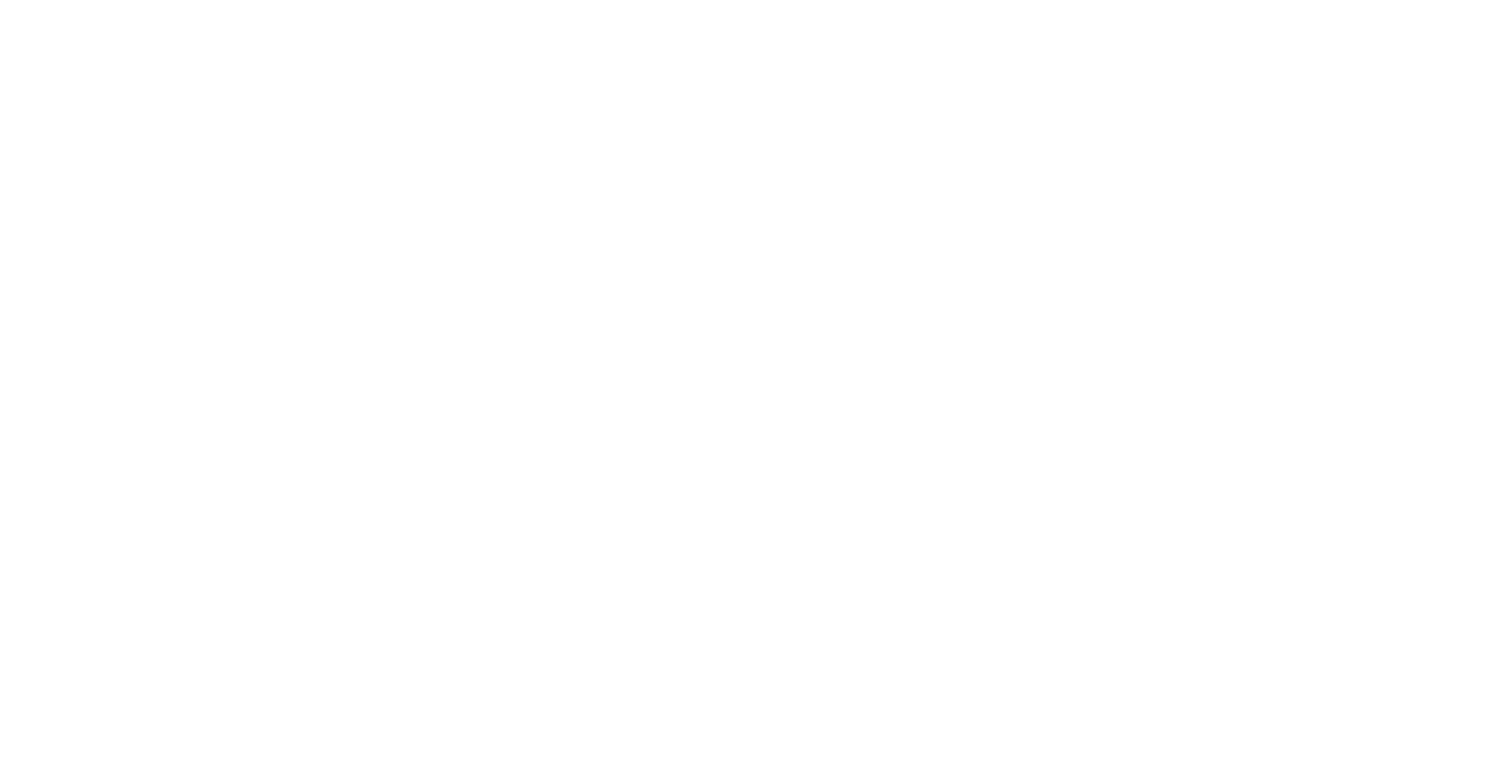
The State and Trends of Australia's Ocean Report was supported by IMOS. IMOS gratefully acknowledges the additional support provided by the Commonwealth Scientific and Industrial Research Organisation (CSIRO).
The State and Trends of Australia's Ocean website is maintained by IMOS.
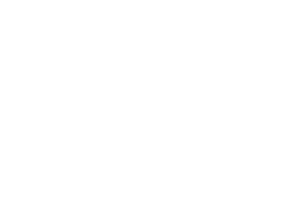
Australia’s Integrated Marine Observing System (IMOS) is enabled by the National Collaborative Research Infrastructure Strategy (NCRIS). It is operated by a consortium of institutions as an unincorporated joint venture, with the University of Tasmania as Lead Agent.
Disclaimer:
You accept all risks and responsibility for losses, damages, costs and other consequences resulting directly or indirectly from using this site and any information or material available from it. While the Integrated Marine Observing System (IMOS) has taken reasonable steps to ensure that the information on this website and related publication is correct, it provides no warranty or guarantee that information provided by the authors is accurate, complete or up-to-date. IMOS does not accept any responsibility or liability for any actions taken as a result of, or in reliance on, information on its website or publication. Users should check with the originating authors to confirm the accuracy of the information before taking any action in reliance on that information.
If you believe any information on this website or in the related publication is inaccurate, out of date or misleading, please bring it to our attention by contacting the authors directly or emailing us at IMOS@imos.org.au
Images and Information:
All information on this website remains the property of those who authored it. All images on this website are licensed through Adobe Stock, Shutterstock, or have permission from the original owner.